God's Particle |
There are three fundamental forces. The most familiar is gravity, which holds humans and other objects to the Earth, makes the Moon go around the Earth thereby leading to tides, lunar phases and eclipses, and causes the Earth to orbit the Sun thereby leading to seasons. Gravity is generated by objects with mass. But because gravity is such a weak force, only bodies of huge mass, such as the Earth and Sun, create a significant effect. In the subatomic world, where protons, neutrons and electrons are extremely light, gravity plays no role.
The second fundamental force is a combination of three forces previously thought to be independent of one another: magnetism, the electric force and the weak subnuclear interaction. The unification of the electric and magnetic forces was achieved in the 19th century, leading to electromagnetism. The electromagnetic force is the source for all macroscopic forces except those created by gravity. Friction, spring forces, air pressure, the forces in collisions, and so on, originate from the electromagnetic force. The weak subnuclear interaction is responsible for certain decays of nuclei and plays a role in generating the energy of the Sun and other stars in their cores. As its name implies, it operates at distances smaller than a nucleus and it is very weak. For this reason, it is difficult to observe. At the end of the 1960's, a theory was proposed that unified the weak subnuclear force with electromagnetism. Experiments in the 1970's and 1980's confirmed the electroweak theory. In 1979, Steven Weinberg, Sheldon Glashow and Abdus Salam received the Nobel Prize in physics for unifying the weak subnuclear interaction with electromagnetism.
The third fundamental force is called the strong nuclear force. It binds three quarks together to from the proton and the neutron. It is also responsible for causing protons and neutrons to stick to one another in a nucleus.
One of the key ideas in physics is that the basic particle forces are generated through the exchange of vector gauge bosons. These are particles that spin with one fundamental unit and incorporate an enormous amount of symmetry. The electromagnetic force is generated when charged particles exchange photons (spin one particles of "light"), the weak subnuclear interactions are generated by exchanging heavy vector bosons known as W's and Z, while the strong force is produced by eight gluons.
The fundamental constituents – quarks and leptons – along with the two fundamental particle interactions – the electroweak interaction and the strong nuclear force constitute The Standard Model of particle physics. Gravity has not been yet incorporated because a good quantum theory of gravitation is not available.
It would seem that scientists know everything there is to know about microscopic matter and its interactions. However, one aspect of The Standard Model has remained a mystery: the mechanism that produces fundamental mass. The weak subnuclear interactions are feeble and short-ranged because the W and Z have very heavy masses of 90 - 100 times the mass of a proton. In contrast, the photon is massless. It is this great mass difference that makes electromagnetism so different from the weak subnuclear force. How are masses for the W and Z created? During the past 30 years, theorists have proposed various mechanisms, of which only experiment can decide which is correct. One way to give masses to the W and Z is to use particles known as Higgs fields. Four such particles are needed. Three are absorbed by the W and Z and one is left over. In this mechanism, there should be one spin zero, electrically neutral particle observed in nature. This is the "God particle," the Higgs particle. The potential break-through discovery at CERN suggests that W and Z masses are created by the Higgs mechanism. The positively charged W, the negatively charged W and the neutral Z obtain mass by respectively absorbing positively charged, negatively charged and neutral Higgses.
The Higgs field also has the ability to generate masses for the quarks and leptons. Thus, if the expected properties of the Higgs field are confirmed, then the origin of all mass will be understood.
The generation of mass proceeds through a process known as spontaneous symmetry breaking. An object has symmetry if rotating it does not change its appearance. For example, if a rod is rotated as indicated in Figure A, its appearance is unchanged. A sphere has even more symmetry than a rod because a sphere can be rotated in many ways without changing its shape.
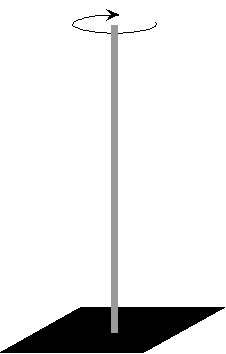
Figure A
Spontaneous symmetry breaking occurs when a system or object naturally looses its rotational invariance. Suppose pressure is exerted on the rod; say by pushing on it with a finger. Then, if sufficient force is applied, the rod will buckle. See Figure B. When a buckled rod is rotated, one can tell that it has been rotated; thus, the symmetry has been broken.
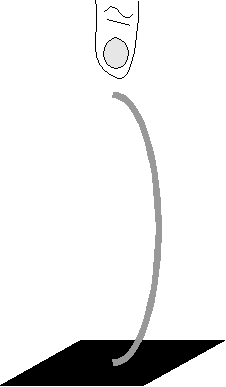
Figure B
By the way, if the force is exerted exactly along the axis of the rod from the top, one might think that it is impossible for the rod to buckle in a particular direction. However, the buckling WILL occur but the direction is unpredictable. This is a feature of spontaneous symmetry breaking. The breaking takes place but one does not know in which direction.
The Higgs field thus acts as the finger in Figure B, applying pressure to the system to cause it to buckle and loose its symmetry. It is a deep result of quantum field theory that when this happens that the W and Z, which are messengers of the symmetries broken by the Higgs, acquire masses. In other words, without spontaneous symmetry breaking the W and Z would be massless.
The Higgs field produces masses for the quarks and the electrically charged leptons through its interactions with these fields. These masses are proportional to the strength with which the Higgs couples to the particles. Because the Higgs interacts most strongly with the top quark, the top quark weighs the most (about 200 times the mass of a proton). The electron interacts very weakly with the Higgs and that is why it is the lightest particle (about 2000 times lighter than a proton). Physicists do not understand why the Higgs couplings differ so greatly, so that even if the CERN experiment is confirmed and the Higgs mechanism is realized, certain features of the Standard Model will remain a mystery.
So if one of the most important scientific discoveries has been made, why has there been so little news about it? The answer is that because of its great importance, experimentalists must be sure of the result before announcing it. There are four experimental detectors at CERN. Of these four, only Aleph is seeing convincing evidence of Higgs production. That detector sees three Higgs-candidate events. Another detector, Delphi, also thinks that it has produced one Higgs in a single positron-electron collision. Although Aleph states that the Higgs has been seen with better than 99% confidence, no strong claims can be made with so few events. CERN has decided to run its LEP experiment an extra month or so to try to produce more Higgs particles. If successful, an important announcement on the Higgs discovery will be made near the end of this year.
No comments:
Post a Comment
Please be nice :)